Applications of zeolite in various fields
For many years, zeolite has been mainly used for blood purification in the medical field. In developed countries such as Europe and the United States, micronized zeolite has been hailed as a "natural medical device" in the medical field.
Because zeolite itself has a regular porous structure and small particle size, it can filter molecules, exchange cations, and adsorb heavy metal substances. Therefore, after zeolite enters the human body, it can adsorb and remove a variety of toxins, radioactive elements and other harmful metabolites in the human body.
In recent years, natural zeolite has been widely used in green building materials, petrochemical industry, soil improvement, sewage treatment, metallurgy, medicine, atomic energy industry and light industry, becoming an important new natural and environmentally friendly material in the national economy. Therefore, the development of natural zeolite and applications are attracting more and more attention.
1. In the petroleum and chemical industries: used as catalytic cracking, hydrocracking in petroleum refining and chemical alienation, reforming, alkylation and disproportionation of petroleum; gas and liquid purification, separation and storage agents; hard water softening and seawater desalination. Agent; special desiccant (dry air, nitrogen, hydrocarbons, etc.).
2. In the light industry: used in papermaking, synthetic rubber, plastics, resins, paint fillers and quality colors, etc. It is used as adsorption separation agent and desiccant in national defense, space technology, ultra-vacuum technology, energy development, electronic industry, etc.
3. In the field of green building materials: This is the largest application field of zeolite. According to statistics, two-fifths of the world's zeolites are used in the building materials industry, which can effectively improve the performance of concrete; or used in wall decoration materials. Zeolites have strong adsorption capabilities and can absorb polar molecules such as H2O, NH3, H2S, CO2, etc. have high affinity and can still be effectively adsorbed even under conditions of low relative humidity, low concentration, and high temperature. 4. In agriculture: Zeolite can be used as a soil conditioner to maintain moisture, fertility, and adjust pH. In the production of chemical fertilizers and pesticides, zeolite can be used as filler and solidification dispersion medium.
5. In terms of environmental protection: Zeolite can be used to treat waste gas and waste water, remove or recover metal ions from waste water and liquid, and remove radioactive pollutants from waste water.
6. In medicine: Zeolite is used to measure the amount of nitrogen in blood and urine. Zeolite has also been developed as a health product for anti-aging and removing heavy metals accumulated in the body.
7. In supply: Zeolite is often used in the refining of sugar.
8. Raw materials for new wall materials (aerated concrete blocks): As solid clay bricks gradually withdraw from the stage, the application proportion of new wall materials has now reached 80%. Wall material supply companies use coal gangue, fly ash, ceramsite, Slag, light industrial waste, heavy construction waste, zeolite, etc. are used as main materials to actively develop new wall materials.
9. In chemical distillation or heating experiments: often used to prevent bumping. There are a large number of small pores in the structure of zeolite, which can be used as condensation nuclei of bubbles to make the reaction liquid boil smoothly. Bisque-fired porcelain pieces broken to the size of rice grains can be used instead.
10. It can be used as a fish and shrimp feed additive in aquaculture, and can also be used as fish pond construction materials to purify water quality. Ammonia filtration for fish hatcheries; biological filter media.
Powder coating particle size control and application
Powder coating is composed of micron particles with a particle size between 10 and 100 μm. Its preparation process and application performance are affected by the particle size, including surface charge, storage stability, powder loading rate during electrostatic spraying, and fluidized bed In-use stability, angle powder application and coating efficiency, etc. Starting from the surface characteristics of particles, the correlation between particle size and surface charge is introduced, which extends to the impact of particle distribution on product characteristics. It also discusses how to achieve a specific particle size distribution in the mechanical crushing and separation process.
In the production process of powder coatings, the particle size is broken into a particle size suitable for coating through a grinding mill for decades. However, the particle size spacing obtained by traditional grinding is usually between 1.8 and 2.0, which reduces the The diameter requires double cyclones to remove fine powder, thereby significantly reducing production efficiency and product yield. Grinding to obtain a narrow particle size distribution while achieving high yield has always been a major challenge in industrial production. In recent years, the particle size optimization grinding unit developed by Jiecheng can effectively adjust the fine powder content <10 μm by optimizing the grinding and classification process, and ensure that no fine powder is produced by repeatedly grinding large particles to the set particle size range. Products with large particle sizes are screened and removed, thereby controlling the particle size distribution within the diameter range of 1.3 to 1.6. At the same time, products with very high particle size concentration are obtained without reducing the yield.
Due to the agglomeration between particles, the smaller the particle size, the larger the void ratio; the wider the particle size distribution range, the packing density tends to become larger due to the filling effect of small particles between large particles. Close packing cannot be achieved with a single particle. Only multiple particle sizes can achieve close packing. Moreover, the greater the difference in particle size, the higher the packing density. When the gap between small particles and large particles is 4 to 5 times, finer particles can be filled. In the gaps of large particles, the shape and filling method of the particles will also affect the packing density. When there are two particle sizes with a quantity ratio of 7:3, or three particle sizes with a quantity ratio of 7:1:2, the entire The system has the highest packing density. Higher bulk density can improve the uniformity of the coating film, thereby achieving excellent leveling effect and gloss.
The grinding equipment that usually grinds powder coatings into suitable particle sizes is an air classification mill (ACM). The principle is that after the flakes enter the main grinding disc of the grinding mill, they are crushed into particles through centrifugal force and the collision with the grinding column of the main grinding mill. Then the inner wall of the grinding body is carried by the air flow to the cyclone separator for particle size classification. The grinder consists of a main grinding mill, an auxiliary grinding mill (classifier), a screen and a cyclone separator. The air volume and screen selection determine the proportion of small particles and large particles; at the same time, the characteristics of the powder coating, the feeding speed, the ambient temperature and humidity and air supply temperature also have a crucial impact on the size of the ground particles.
The currently industrialized particle size optimization mill can effectively reduce the formation of fine powder by changing the balance of the air inlet and outlet systems in the system, and obtain products with high particle size concentration. At the same time, the median particle size can be between 15 and 60 Adjusted within the μm range, it can produce products with normal particle sizes as well as thin-coated powders with a median particle size of 15 to 25 μm.
Applications of ground fiberglass
Ground glass fiber is made by grinding glass fiber raw strands using crushing equipment such as a hammer mill or ball mill. The average length of the fiber is 30 to 100 microns. When observed under a microscope, its cross-sectional area is cylindrical. Ground glass fiber in my country is usually calibrated by fiber length and fiber diameter. For example, EMF-200 refers to ground fibers with an average diameter of 7.5 microns and an average length of 80 to 110 microns.
At present, ground glass fiber is mainly used as high-performance friction material in my country. The traditional filler of friction materials is asbestos. However, it has been reported abroad that asbestos is a carcinogen. The international market has boycotted friction materials containing asbestos in recent years, providing a broad market for grinding glass fibers.
The ground glass fiber used as friction material undergoes surface chemical treatment to accelerate the penetration of resin and meet special molding performance and product performance requirements. Its specifications include EMF-200, EMF-250 and EMF-300, and the corresponding fiber length fluctuation ranges are 110-80 microns, 80-50 microns and 50-30 microns.
The friction material added with ground glass fiber not only has a high friction coefficient, but also has durability and thermal stability. When it comes into frictional contact with the close parts (such as the rotor), it only produces slight noise and causes wear of the rubbed parts. Volume is reduced to a minimum.
This high-performance friction material can be widely used as brake pads and clutch plates for automobiles, brake shoes for passenger and freight vehicles, railway locomotives and various drilling rigs, friction blocks for stamping equipment and engineering and mining machinery, and cones for lifting machinery. Shaped brake rings, etc.
Ground glass fiber can also be used as functional filler in ABS plastic to modify ABS plastic to meet the requirements of plastic processing and product application performance. When a factory was producing parts such as the program controller bottom plate and cover plate of a fully automatic washing machine, because they were made of pure ABS plastic, the bottom plate and cover plate were seriously warped and deformed, the dimensions of the parts were unstable, and the screw holes slipped. teeth, causing many finished products to be scrapped because they could not be assembled. Later, ground glass fiber was used to fill ABS plastic to modify the plastic: the shrinkage rate was reduced from the original 1% to 2% to 0.4% to 0.5%. When tightening self-tapping screws, the teeth will not slip or crack, and it will be manufactured at the same time. The board surface and parts are smooth, stiff and not warped, and the plastic processing performance is good. In addition, adding ground glass fiber to the laminate can improve the crack resistance and wear resistance of the laminate, reduce the shrinkage of the laminate, and improve the lamellar strength. At the same time, it also plays a certain role in improving the bending elastic modulus of laminates and compressing elastic molds. When the resin mixture with ground glass fiber is added to the mold, it can smooth the outer surface defects, round the edges and corners of the mold structure, and also round the reinforcing ribs covered with glass cloth that are pre-connected to the outside of the mold structure.
After the resin reinforced with ground glass fiber is cured, the hardness of the product is higher, and its thermal expansion performance is similar to that of hand lay-up FRP reinforced with glass cloth, so the product is less likely to crack.
The simultaneous use of surface-active components and ground glass fibers in the resin system can improve its wet strength performance, reduce water absorption, and increase the retention rate of wet strength.
10 Major Application Fields of Silica Powder
Microsilica powder is a silica powder made from crystalline quartz, fused quartz, etc., and processed through grinding, precision classification, impurity removal and other processes. It is widely used in copper-clad laminates, epoxy plastic sealants, electrical insulation materials, rubber, Plastics, coatings, adhesives, artificial stone, honeycomb ceramics, cosmetics and other fields.
1. Copper clad laminate
At present, silica powder used in copper-clad laminates can be divided into crystalline silica powder, fused silica powder, spherical silica powder and composite silica powder. The filling weight ratio of resin in the copper clad laminate is about 50%, and the filling rate of silica powder in the resin is generally 30%, that is, the filling weight ratio of silica powder in the copper clad laminate is about 15%.
2. Epoxy plastic sealing compound
Microsilica powder is the most important filler in epoxy molding compound (EMC), accounting for about 60%-90%. The performance improvement of epoxy molding compound needs to be achieved by improving the performance of silica powder. Therefore, the particle size, purity and sphericity of silica powder are important. degree has higher requirements.
3. Electrical insulation materials
Microsilica powder is used as epoxy resin insulating filler for electrical insulation products. It can effectively reduce the linear expansion coefficient of the cured product and the shrinkage rate during the curing process, reduce internal stress, and improve the mechanical strength of the insulating material, thereby effectively improving and improving the insulating material. mechanical and electrical properties.
4. Rubber
Silica powder has the advantages of small particle size, large specific surface area, good heat resistance and wear resistance, and can improve the wear resistance, tensile strength and modulus, high tear and other properties of rubber composite materials. However, the surface of silica powder contains a large amount of If the acidic silanol groups are not modified, the silica powder will be unevenly dispersed in the rubber, and the acidic groups will easily react with alkaline accelerators, prolonging the vulcanization time of the rubber composite.
5. Plastic
Microsilica powder can be used as a filler in polyethylene (PE), polyvinyl chloride (PVC), polypropylene (PP), polyphenylene ether (PPO) and other materials in the process of making plastics. It is widely used in construction, automobiles, electronic communications , insulation materials, agriculture, daily necessities, national defense and military industry and many other fields.
6. Paint
Microsilica powder can be used as a filler in the coating industry. It can not only reduce the cost of preparing coatings, but also improve the high temperature resistance, acid and alkali resistance, wear resistance, weather resistance and other properties of coatings. It can be widely used in building materials, automobiles, pipes, hardware, etc. Household appliances and other fields.
7. Adhesive
As an inorganic functional filling material, silicone powder can effectively reduce the linear expansion coefficient of the cured product and the shrinkage during curing when filled in the adhesive resin, improve the mechanical strength of the adhesive, improve heat resistance, permeability and heat dissipation performance, thereby improving the adhesion knotting and sealing effect.
The particle size distribution of silica powder will affect the viscosity and settling properties of the adhesive, thereby affecting the processability of the adhesive and the linear expansion coefficient after curing.
8. Artificial quartz stone
When silica powder is used as a filler in artificial quartz slabs, it can not only reduce the consumption of unsaturated resin, but also improve the wear resistance, acid and alkali resistance, mechanical strength and other properties of artificial quartz slabs.
9. Automobile honeycomb ceramics
The automotive exhaust filter DPF (Diesel Particulate Filter) made of honeycomb ceramic carrier for automobile exhaust purification and cordierite material for diesel engine exhaust purification is made of alumina, silica powder and other materials through mixing, extrusion molding, drying, sintering, etc.
10. Cosmetics
Spherical silica powder has good fluidity and large specific surface area, making it used in cosmetics such as lipstick, powder cake, and foundation cream.
Different application fields of silica powder have different quality requirements. The application research of silica powder will mainly focus on high-tech fields such as high-end copper-clad laminates, high-end coatings, high-performance adhesives, and insulating materials produced using spherical silica powder as raw materials. Refinement and functionality Specialization will be the mainstream direction of silica powder application in the future.
New thermal management materials continue to develop
Thermal conductive material is a material that improves heat distribution and heat conduction efficiency in heat conduction and is used to ensure the reliability and service life of electronic equipment systems. According to its application scenarios and morphological properties, it mainly includes graphite heat dissipation film, thermal conductive heat dissipation materials (heat pipes, vapor chambers, etc.) and thermal conductive interface materials (such as thermal conductive silicone grease, thermal conductive gel, etc.).
The industrial development of thermally conductive materials can be traced back to the 1950s, when thermally conductive materials were mainly aluminum and copper; from the 1960s to the 1970s, silicone materials began to develop rapidly and heat pipes appeared. From the 1970s to the early 21st century, graphite materials developed rapidly and were widely used. Since then, with the development of new industries such as 5G and power batteries, the demand for thermal conductivity has surged, and new thermal management materials have continued to develop.
Thick graphite film
Natural graphite film is the first graphite-based heat distribution material and the earliest used heat distribution material. High-carbon flake graphite can obtain natural graphite film through chemical treatment and high-temperature expansion rolling. The manufacturing process is simple, and my country has abundant natural graphite reserves and outstanding cost advantages. The problem with natural graphite film lies in the following two points: First, as a natural product, its sheets are prone to structural defects, which will affect the local heat distribution performance; second, although the lateral thermal conductivity of natural graphite has exceeded that of most materials, However, its longitudinal thermal conductivity is not outstanding enough and it is mainly used in low-end product fields.
Graphene
Graphene is a new heat-distributing material, known as the "hexagonal warrior", with strong lateral thermal conductivity and flexibility. Graphene refers to a single layer of carbon atoms. Its theoretical thermal conductivity is as high as 5300W/m·K, making it one of the substances with the highest thermal conductivity so far. With the continuous enhancement of the performance of electronic products, the increasing demand for heat equalization has driven the use of graphene membranes. In addition to high thermal conductivity, the flexibility of graphene films is also an important property.
Ultra-thin heat pipe
The heat pipe has rapid temperature equalization characteristics and is composed of an outer hollow metal tube and an inner phase-changeable liquid. Its working principle is to rapidly equalize the temperature of the surface of the tube through the continuous circulation of liquid and vapor two-phase changes in the hollow metal tube cavity. Heat pipes are commonly used in various heat exchangers, coolers, etc., and are mainly responsible for rapid heat conduction. They are currently the most common and efficient heat-conducting element in the heat dissipation devices of electronic products.
Ultra-thin vapor chamber
Vapor chambers are high-end thermal devices and are mainly used in thickness- or weight-sensitive equipment. The vapor chamber is generally composed of external copper and internal phase-changeable condensate. Its structure and thermal soaking principle are similar to those of a heat pipe. The difference is that the vapor chamber takes on a two-dimensional plate shape. Through the four steps of conduction, evaporation, convection and solidification, the heat released by the point heat source is evenly distributed on the entire plane. The heat equalizing effect exceeds that of graphite-based materials.
Hybrid Filled Thermal Interface Materials
Thermal interface materials generally consist of two parts: matrix material and filler. The base material is mainly used to ensure that the thermal interface material can cover all locations where air gaps exist, and it is mainly made of fluid polymers. Fillers are made of various materials with high thermal conductivity, such as metals and metal oxides, nitrides, carbides, etc., to improve heat transfer efficiency.
Composite thermally conductive phase change materials
Thermal conductive phase change materials are mainly used in high-performance devices that require small thermal resistance and high thermal conductivity efficiency, with high reliability and strong safety. Its working principle is to use the phase change process to conduct heat. When the temperature reaches the phase change point, the thermal conductive phase material will undergo a phase change, changing from a solid state to a fluid state, and flows into the irregular gap between the heating element and the radiator under pressure.
The importance of powders to advanced ceramics
The importance of powders to advanced ceramics is directly reflected in people's definition of advanced ceramics.
The general definition of advanced ceramics is: using high-purity, ultra-fine artificially synthesized or selected inorganic compounds as raw materials, having precise chemical composition, precise manufacturing and processing technology and structural design, and having excellent mechanical, acoustic, optical and thermal properties. Ceramics with , electrical, biological and other properties are oxides or non-oxides composed of metallic elements (Al, Zr, Ca, etc.) and non-metallic elements (O, C, Si, B, etc.). They are composed of ionic bonds and covalent bonds. Bonded jointly bonded ceramic materials.
In terms of chemical composition, two aspects are generally pursued: high purity and precise ratio.
In terms of high purity. The presence of impurities can sometimes seriously affect the performance of products. For example, impurities such as silicon, calcium, iron, sodium, and potassium often exist in high-purity alumina. The presence of iron impurities will make the sintered material black and dark; sodium and potassium impurities will affect the electrical properties of the material, causing its electrical properties to deteriorate; and the remaining two impurities will cause the material's grains to grow abnormally during the sintering process. In terms of transparent ceramics, the impact of impurities is even greater. The presence of impurities in ceramic powder will directly declare the "blindness" of transparent ceramics. This is because impurities, as the second phase, are very different from the optical properties of the ceramic body material, often causing Scattering and absorption centers will greatly reduce the light transmittance of ceramics. In nitride ceramics such as silicon nitride and aluminum nitride, the presence of oxygen impurities can lead to a decrease in thermal conductivity.
In terms of ratio. In ceramic production formulas, most of the time there is no need for an extremely "high-purity" single component, but some auxiliary materials, such as sintering aids, are often added. In this case, accurate proportioning is the most basic requirement, because different chemical compositions and contents will have a decisive impact on the performance of the product.
Phase composition
Generally, the powder is required to have the same physical phase as the ceramic product as much as possible, and phase change is not expected to occur during the sintering process. Although sometimes phase change can indeed promote the densification of ceramics, in most cases, the occurrence of phase change is not conducive to the sintering of ceramics.
Particle size and morphology
Generally speaking, the finer the particles, the better. Because according to the existing sintering theory, the speed of body density is inversely proportional to the size of the powder (or its size to a certain power). The smaller the particles, the more conducive to sintering. For example, due to its high specific surface area, ultrafine aluminum nitride powder will increase the sintering driving force during the sintering process and accelerate the sintering process.
The better fluidity of ceramic powder with regular shape will have a positive impact on subsequent molding and sintering. The granulation process is to allow the powder to form a spherical shape under the action of the binder, which also indirectly shows that Spherical ceramic powder plays a positive role in improving the density of ceramics during the molding and sintering processes.
Uniformity
The uniformity of powder is easily overlooked, but in fact its importance is more important than the previous aspects. In other words, the performance of the previous aspects is very important to see its uniformity.
The same goes for particle size. Fine particle size is important, but if the average particle size is only fine and the distribution is uneven or very wide, it will be extremely detrimental to the sintering of ceramics. Because particles of different sizes have different sintering speeds, areas with coarser particles are not likely to be dense. At the same time, coarse particles may also become the nucleus for abnormal grain growth. Finally, the ceramic not only needs to be densified at a higher temperature, but also has an uneven microstructure, seriously affecting its performance.
Process Difficulties of Transparent Ceramics
As a high-tech material, transparent ceramics have broad application prospects in the fields of optics, electronics, aerospace and other fields. However, there are many difficulties in the preparation process of transparent ceramics, which are mainly reflected in the following aspects:
1. High-temperature smelting: The preparation of transparent ceramics requires the raw materials to be smelted into a transparent glass phase at high temperatures, usually as high as 1700°C or above. In this process, temperature and melting time need to be controlled to avoid the generation of impurities and crystallization, while ensuring the uniformity and transparency of the glass phase.
2. Removal of bubbles: During the preparation process of transparent ceramics, the generation of bubbles is a common problem. These bubbles can form defects inside the ceramic, affecting its optical properties and mechanical strength. In order to remove bubbles, special degassing processes and equipment are required, such as vacuum degassing, inert gas protection, etc. In the preparation process of transparent ceramics, the removal of air bubbles is a key step.
3. Precisely control the composition: The composition of transparent ceramics has an important impact on its optical and mechanical properties. In order to prepare high-quality transparent ceramics, the ingredient ratio and purity of the raw materials need to be precisely controlled to ensure they remain consistent throughout the preparation process.
4. Sintering temperature: The sintering temperature of transparent ceramics is crucial to its densification and optical properties. If the temperature is too high, the ceramic will crystallize or produce other impurities. If the temperature is too low, the sintering will be incomplete or the density will be insufficient.
5. Dimensional accuracy: Transparent ceramics need to maintain high dimensional accuracy during the preparation process to ensure their optical and mechanical properties. This requires precise control of the preparation process, such as mold design and processing, molding pressure, etc. At the same time, the shrinkage rate of ceramics needs to be controlled during the sintering process to ensure the dimensional accuracy of the final product.
Particle size of raw materials: The particle size of raw materials for transparent ceramics has a direct impact on its dimensional accuracy. If the particle size distribution of the raw materials is uneven, the size of the ceramic products will be unstable. Therefore, raw materials with uniform particle size distribution and suitable particle size should be selected during production and strictly screened.
Molding process: The molding process is a key link that affects the dimensional accuracy of transparent ceramics. Different molding methods (such as die-casting, extrusion, grouting, etc.) have different effects on dimensional accuracy. When selecting the molding process, a reasonable selection should be made based on the product shape, dimensional accuracy requirements and production batch size.
Firing system: Firing is an important part of the production of transparent ceramics. A suitable firing system is crucial to improving the dimensional accuracy of transparent ceramics. Temperature curve, firing time, firing atmosphere and other factors will affect the dimensional accuracy of transparent ceramics. During production, a reasonable firing system should be formulated based on the actual situation, and the firing process should be monitored in real time to ensure the stable execution of the firing system.
Equipment and tools: The accuracy of production equipment and tools will also affect the dimensional accuracy of transparent ceramics. For example, the accuracy of molds, cutting tools, etc. directly affects the size of ceramic products.
Quality inspection and control: In order to ensure the dimensional accuracy of transparent ceramics, a complete quality inspection and control system should be established during the production process.
6. Cooling rate: During the preparation process of transparent ceramics, the cooling rate has an important impact on its internal structure. Cooling too quickly may cause internal stress or cracks, while cooling too slowly may cause crystallization or other impurities.
There are many difficulties in the preparation process of transparent ceramics, and multiple aspects such as raw materials, processes, equipment, and preparation environment need to be comprehensively considered. Only through continuous technological innovation and accumulation of practical experience can high-quality transparent ceramic materials be prepared.
Innovative applications of silicon carbide ceramics and 3D printing technology
Ceramic components for semiconductor equipment have high requirements in terms of material purity, dimensional accuracy, mechanical properties, thermal properties, and electrical properties. Silicon carbide ceramics are a type of material that has been proven by the market and has excellent performance for semiconductor equipment components. It is widely used in ceramic manipulators (stiffness, wear resistance), crystal boats (purity, high-temperature mechanical properties), and cold plates (thermal conductivity, rigidity). ), workbench (dimensional accuracy, execution stability) and other components have important applications.
However, in the face of market demands for large size, complex structure, short manufacturing cycle, high stability, and low cost, the traditional manufacturing process of silicon carbide ceramics has encountered bottlenecks. Use additive manufacturing technology to achieve a breakthrough in the manufacturing technology of ceramic components for silicon carbide ceramic semiconductor equipment.
3D printed semiconductor equipment silicon carbide ceramic components, according to different size, shape and purpose requirements, are processed through 3D printing, reaction sintering and finishing to obtain high purity, high temperature qualitative, high thermal conductivity, high temperature resistance, friction and wear resistance properties Excellent products that can meet the needs of many application scenarios for ceramic components in semiconductor equipment. This series of products has a short production cycle, is standardized and mass-produced, and can achieve differentiated structural design that is more suitable for efficient and high-quality production in the semiconductor industry.
Through 3D printing technology, we can design and manufacture silicon carbide ceramic parts with high purity, high temperature stability, high thermal conductivity, high temperature resistance, and excellent wear resistance according to different size, shape and functional requirements. These components not only meet the needs of diverse applications, but also have short production cycles, are standardized, can be mass-produced, can realize differentiated structural design, and are more suitable for efficient and high-quality production needs of various industries.
In addition, based on PEP (Powder Extrusion Printing) technology, we can manufacture ceramic and metal products with complex structures through "3D printing + powder metallurgy". These products have consistent and excellent performance, which can effectively reduce the production cycle and production cost.
The space mirror is a large-sized, lightweight, integrated design near-net-shape silicon carbide ceramic complex structural component manufactured by Sublimation 3D based on the PEP process. The density can be as high as 99% and the mechanical properties are stable. It has effectively promoted the development of remote sensing satellites and the construction of space infrastructure, which can significantly reduce costs and shorten the R&D and production cycle. There is a huge market space for remote sensing satellites, which are the fastest growing in the commercial aerospace field.
Silicon carbide ceramic material
With the development of science and technology, especially the rapid development of energy and space technology, materials are often required to have superior properties such as high temperature resistance, corrosion resistance, and wear resistance in order to be used in harsh working environments. Special ceramic materials have become cutting-edge due to their excellent properties such as strong oxidation resistance, good wear resistance, high hardness, good thermal stability, high high-temperature strength, small thermal expansion coefficient, high thermal conductivity, and resistance to thermal shock and chemical corrosion. An important part of science that is universally valued.
Silicon carbide ceramics are a new material that has only begun to develop in the past twenty years. However, due to its particularly excellent high strength, high hardness, corrosion resistance, and high temperature resistance, it has been quickly developed and used in petrochemical and metallurgical industries. Machinery, aerospace, microelectronics, automobiles, steel and other fields, and increasingly show advantages that other special ceramics cannot match.
The rapid development of modern national defense, nuclear energy and space technology, as well as the automobile industry and marine engineering, has placed increasingly high demands on materials such as rocket combustion chamber linings, aircraft turbine engine blades, nuclear reactor structural components, high-speed pneumatic bearings and mechanical seal parts. A variety of new high-performance structural materials need to be developed.
Silicon carbide (SiC) ceramics have excellent properties such as high high-temperature strength, strong oxidation resistance, good wear resistance, good thermal stability, small thermal expansion coefficient, high thermal conductivity, high hardness, and resistance to thermal shock and chemical corrosion. Therefore, it has shown its talents in many fields and is increasingly valued by people.
For example,
SiC ceramics have been widely used in various corrosion-resistant containers and pipes in the petrochemical industry;
It has been successfully used as various bearings, cutting tools and mechanical seal components in the machinery industry;
It is also considered the most promising candidate material in the aerospace and automotive industries for future manufacturing of gas turbines, rocket nozzles and engine components.
Silicon carbide ceramic materials have excellent properties such as high high-temperature strength, strong high-temperature oxidation resistance, good wear resistance, good thermal stability, small thermal expansion coefficient, high thermal conductivity, high hardness, thermal shock resistance and chemical corrosion resistance. It is increasingly widely used in automobiles, mechanical and chemical industry, environmental protection, space technology, information electronics, energy and other fields. It has become an irreplaceable structural ceramic with excellent performance in many industrial fields.
Main application areas of SiC ceramics
(1)Abrasive (2) Refractory materials (3)Deoxidizer (4)Military aspect (5) Electricians and electricians (6) Wear-resistant and high-temperature parts (7) Application of silicon carbide ceramics in the preparation of new energy materials (8) The preferred material for precision ceramic components used in photolithography machines (9) Filtration application of silicon carbide ceramics
7 categories of dry classification equipment and their operating principles
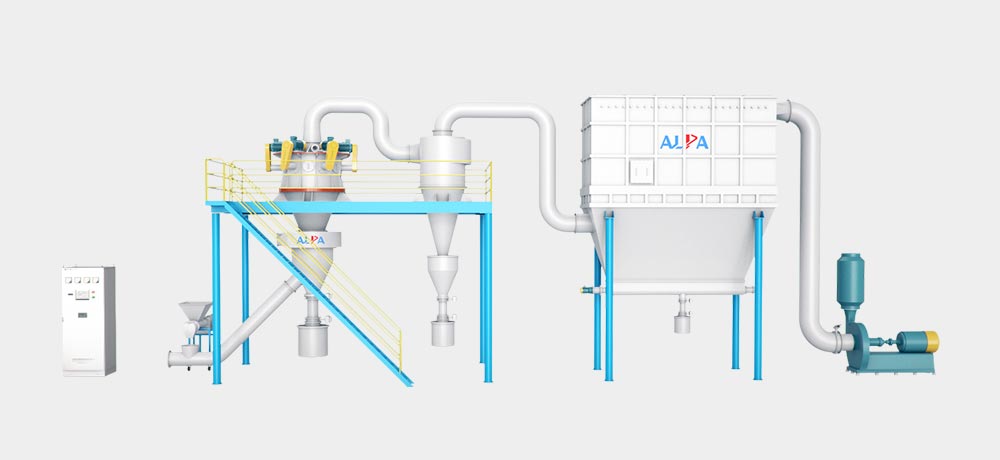
The function of classification is to control the progress of the crushing process and the particle size of the final product. Dry classification is a classification achieved with gas (usually air) as the medium. It is used in water shortage and arid areas, and when the process does not allow the presence of water. , dry grading is the only option. In severe cold areas, the application of dry grading is also not affected. Dry classification saves a lot of water and eliminates the problem of subsequent dehydration in wet classification. It is an effective energy-saving classification method.
Common dry classification equipment includes double impeller air classifier, O-Sepa vortex classifier, cyclone separator, turbine classifier, gravity sedimentation classifier, inertial classifier and jet classifier.
1. Double impeller air classifier
The double-impeller air classifier uses the principles of gravity sedimentation and centrifugal sedimentation to classify, and the product particle size can be as fine as -40 μm.
2. O-Sepa type eddy current classifier
The main structure of the machine includes a spreading plate, impeller, primary air duct, secondary air duct, tertiary air duct, guide blades and shell, etc.
3. Cyclone separator
The cyclone separator is a typical dry centrifugal sedimentation and classification equipment. Its main body consists of an upper cylinder and a lower truncated cone. A core tube is inserted along the central axis from top to bottom at the top of the cylinder, and there is a coarse product outlet at the bottom of the truncated cone. The feed material enters tangentially from the upper part of the cylinder near the outer circumference with the air flow, and is restricted by the shape of the classification chamber to form a swirling motion. The material particles produce radial centrifugal sedimentation motion in the air flow. Coarse particles centrifugally settle at a faster speed, move closer to the cylinder wall, and then slide along the cylinder wall and are discharged from the bottom. The fine particles have a slow centrifugal sedimentation speed, are suspended close to the axis, and then enter the core tube with the air flow and are discharged upward. There are many improved products in practical applications to adapt to different grading requirements and obtain higher grading performance. The classification particle size of the cyclone separator is related to its specification (cylinder diameter). The smaller the specification, the finer the classification particle size.
4. Turbine classifier
Turbine classifier is one of the most widely used dry ultra-fine classification equipment at present. It utilizes the principle of centrifugal sedimentation classification. Its main working component is the turbine (grading wheel), which is equipped with many blades to form a radial gap.
5. Dry gravity sedimentation classification equipment
The main dry gravity sedimentation classification equipment includes horizontal flow type, vertical flow type and meandering flow type gravity classifiers, etc., all of which are used in the ultra-fine stage.
6. Dry inertia classification equipment
The main dry inertial classification equipment includes linear, curved, louver and K-type inertial classifiers, with cut-point particle sizes ranging from 0.5 to 50 μm.
7. Jet classifier
Jet classifier is a dry ultra-fine classification equipment that utilizes jet technology, inertia principle and Coanda effect. Jet technology is used for feeding materials, which allows the feed particles to obtain the necessary inlet velocity and allows the air flow to better produce a Coanda effect. The Coanda effect is when there is surface friction between a fluid (liquid or gas) and the surface of the object it flows through, causing the fluid to slow down. As long as the curvature of the surface of the object is not too large, according to Bernoulli's principle in fluid mechanics, the slowdown in flow speed will cause the fluid to be adsorbed on the surface of the object.